Last week a paper that I co-authored was published in Science! Here is a link to the paper, Origin and hysteresis of lithium compositional spatiodynamics within battery primary particles, and here are several press releases related to the research:
Although the major findings in this work were accomplished by experimental collaborators at Stanford and LBNL, the motivation for the experiments came in part from my postdoc work, where we simulated the heterogeneity of lithium in battery nanoparticles and made several controversial predictions. We calculated that the properties of individual nanoparticles should be significantly different from the measured properties of battery electrodes, which are comprised of billions of particles. Thus the challenge was to build a battery out of a single nanoparticle and measure its properties.
It took several years, but this paper conclusively verifies the prediction of a heterogeneous reaction rate in LiFePO4 with a beautiful set of experiments. Our colleagues at Stanford made a rechargeable battery out of a few nanoparticles, and invented a way to image the chemical composition of the particles as they were cycled in a liquid electrolyte (inside a synchrotron). Below is the first ever video of LiFePO4 nanoparticles being charged and discharged.
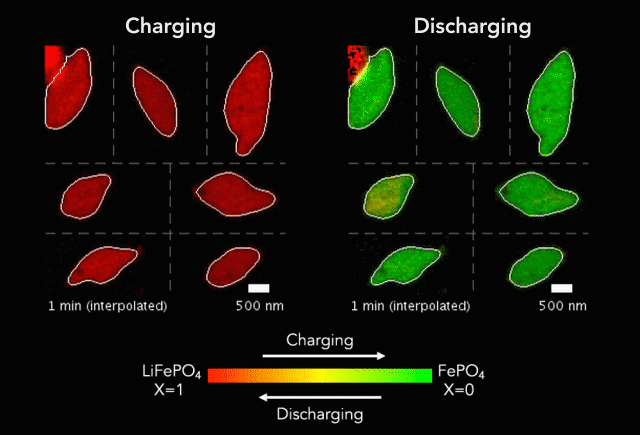